UC Berkeley physicists immobilized small clusters of cesium atoms (pink spheres) in a vertical vacuum chamber, then split each atom into a quantum state in which half of the atom was closer to the mass of tungsten (the shiny cylinder) than the other half (the split sphere under the tungsten). By measuring the phase difference between the two halves of the atomic wave function, they were able to calculate the difference in gravitational attraction between the two parts of the atom that matched what would be expected from Newtonian gravity. Credit: Cristian Panda/UC Berkeley
The experiment captures free-falling atoms to look for gravitational anomalies caused by the universe’s missing energy.
UC Berkeley researchers have increased the accuracy of gravity experiments using an atom an interferometer combined with an optical grating, greatly increasing the time that atoms can be held in free fall. Despite the fact that deviations from Newtonian gravity have not yet been found, these advances could potentially reveal new quantum aspects of gravity and test theories about exotic particles such as chameleons or symmetrons.
Twenty-six years ago, physicists discovered dark energy—a mysterious force that is pushing the universe apart at ever-increasing speeds. Since then, scientists have been searching for a new and exotic particle causing the expansion.
We push the boundaries of this search, University of California, Berkeley physicists have now set up the most precise experiment to date to look for tiny deviations from the accepted theory of gravity that could be evidence for such a particle, which theorists have dubbed a chameleon or symmetron.
The experiment, which combines an atom interferometer for precise gravity measurements with an optical grating to hold atoms in place, allowed researchers to freeze freely falling atoms for seconds instead of milliseconds to look for gravitational effects, beating the current most accurate measurements by a factor of five.
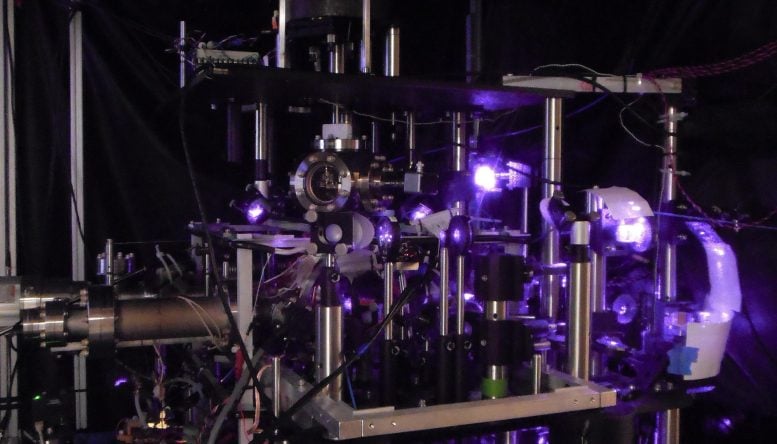
The violet glow of the infrared laser illuminates the optical bench used in the experiment. A laser is used to precisely control the quantum states of cesium atoms in a vacuum chamber. Credit: Holger Müller lab
Exploring the quantum nature of gravity
Although the researchers found no deviation from what the theory proposed by Isaac Newton 400 years ago predicts, the expected improvements in the precision of the experiment could eventually yield evidence that supports or refutes theories of a hypothetical fifth force mediated by chameleons or symmetrons. .
The lattice atom interferometer’s ability to hold atoms for up to 70 seconds — and potentially 10 times longer — also opens up the possibility of probing gravity at the quantum level, said Holger Müller, a professor of physics at UC Berkeley. While physicists have well-tested theories describing the quantum nature of three of the four forces of nature—electromagnetism and the strong and weak forces—the quantum nature of gravity has never been proven.
“Most theorists probably agree that gravity is quantum. But no one has ever seen such an experimental signature,” said Müller. “It’s very hard to even know if gravity is quantum, but if we could hold our atoms 20 or 30 times longer than anyone else, because our sensitivity increases with the second or fourth power of the retention time, we might have a 400 to 800,000 times better chance to find experimental evidence that gravity is indeed quantum mechanical.”
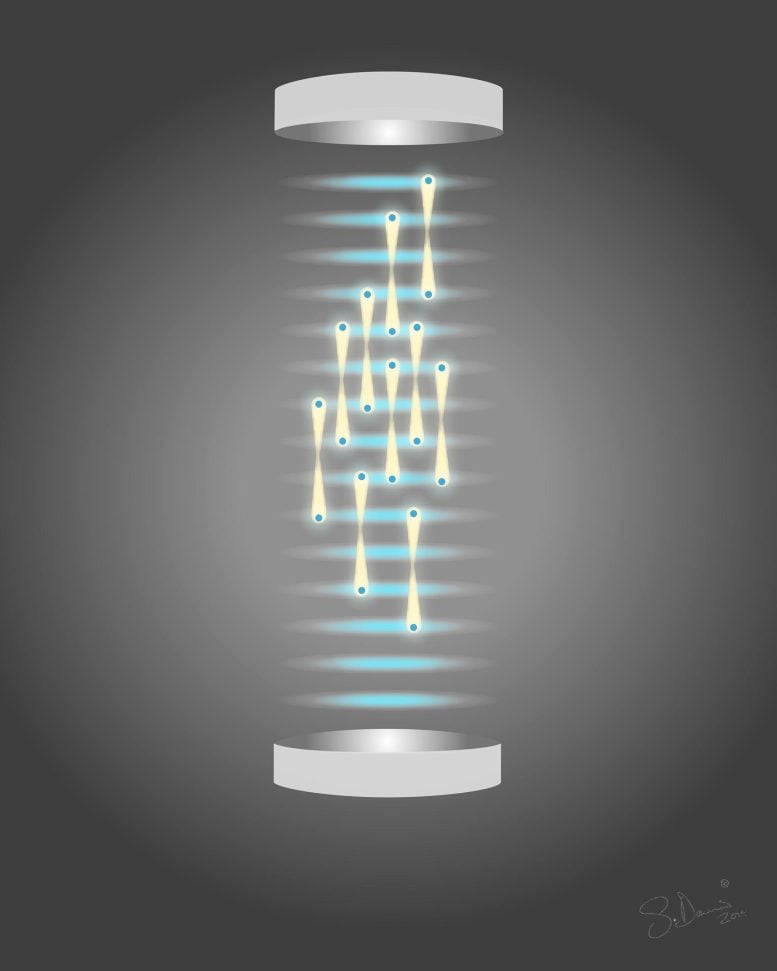
The optical grating captures groups of atoms (blue disks) in a regular array, so they can be studied for more than a minute inside the grating atom interferometer. Individual atoms (blue dots) are located in quantum spatial superposition, i.e. in two lattice layers at once, indicated by elongated yellow bands. Credit: Sarah Davis
Applications and future directions in quantum sensing
In addition to precise gravity measurements, other applications of the lattice atom interferometer include quantum sensing.
Atomic interferometry is particularly sensitive to gravity or inertial effects. You can build gyroscopes and accelerometers,” said postdoctoral researcher Cristian Panda of UC Berkeley, who is the first author of the gravity measurement paper to be published this week in the journal. Nature and is co-authored by Müller. “But this gives a new direction to atom interferometry, where quantum sensing of gravity, acceleration and rotation can be done with atoms held in optical lattices in a compact package that is immune to environmental imperfections or noise.”
Because the optical grating holds the atoms firmly in place, the grating atom interferometer could also work at sea, where sensitive gravity measurements are used to map the geology of the ocean floor.
Insights into dark energy and the chameleon particle
Dark energy was discovered in 1998 by two teams of scientists: a group of physicists based at Lawrence Berkeley National Laboratory, led by Saul Perlmutter, now a professor of physics at UC Berkeley, and a group of astronomers that included UC Berkeley postdoctoral fellow Adam Riess. The two shared the 2011 Nobel Prize in Physics for this discovery.
The knowledge that the universe is expanding faster than it should comes from observing distant supernovae and using them to measure cosmic distances. Despite much speculation by theorists about what actually separates space, dark energy remains a mystery—a big puzzle, since about 70% of all matter and energy in the universe is in the form of dark energy.
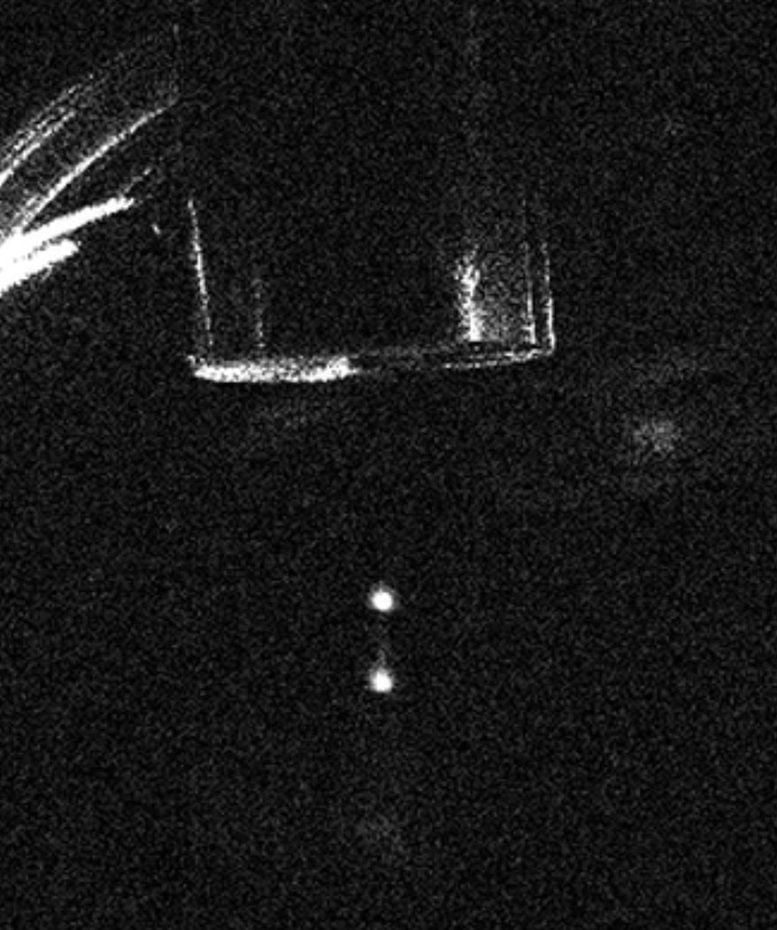
This photo shows clusters of about 10,000 cesium atoms floating in a vacuum chamber, levitated by crossed laser beams that form a stable optical lattice. The cylindrical tungsten weight and its support can be seen above. Credit: Cristian Panda, UC Berkeley
One theory says that dark energy is just the vacuum energy of the universe. Another is that it is an energy field called a quintessence that changes in time and space.
Another proposal is that dark energy is a fifth force much weaker than gravity and is mediated by a particle that exerts a repulsive force that varies with the density of the surrounding matter. In the void of space, it would exert a repulsive force at great distances, capable of compressing space. In a laboratory on Earth, where matter is all around to protect it, the particle would have an extremely short range.
This particle has been called a chameleon, as if hiding in plain sight.
Advances in atomic interferometry techniques
In 2015, Müller modified an atom interferometer to search for evidence of chameleons using cesium atoms released into a vacuum chamber that mimics the emptiness of space. During the 10 to 20 milliseconds it took the atoms to rise and fall above the heavy aluminum sphere, he and his team detected no deviation from what would be expected from the normal gravitational attraction of the sphere and Earth.
The key to using free-falling atoms to test gravity is the ability to excite each atom into a quantum superposition of two states, each with a slightly different momentum that carries them different distances from a heavy tungsten weight hanging overhead. The higher momentum, higher altitude state experiences a greater gravitational pull on the tungsten and changes its phase. When the atom’s wave function collapses, the phase difference between the two parts of the mass wave reveals the difference in gravitational attraction between them.
“Atomic interferometry is the art and science of using the quantum properties of a particle, that is, the fact that it is both a particle and a wave. We split the wave so that the particle takes two paths at the same time, and then cancel them out at the end,” said Müller. “Waves can either be in phase and add up, or they can be out of phase and cancel each other out.” The trick is that whether they are in phase or out of phase depends very sensitively on some quantity you might want to measure, such as acceleration, gravity, rotation, or fundamental constants.
Expanding the boundaries of experimental physics
In 2019, Müller and his colleagues added an optical lattice that keeps atoms close to the tungsten mass for a much longer time—a staggering 20 seconds—to increase the effect of gravity on the phase. The optical grating uses two crossed laser beams to create a grid-like array of stable sites for clustering atoms levitating in a vacuum. But was 20 seconds the limit, he wondered?
During the height COVID 19 Panda worked tirelessly to increase the dwell time, systematically correcting the list of 40 possible obstacles, until it was determined that the fluctuating tilt of the laser beam caused by vibrations was the main limitation. By stabilizing the beam in the resonance chamber and adjusting the temperature to be slightly lower – in this case less than a millionth of a Kelvin higher absolute zeroor a billion times colder than room temperature—he was able to extend the dwell time to 70 seconds.
He and Müller published these results in the June 11, 2024 issue Natural physics.
Gravitational entanglement
In the newly announced gravity experiment, Panda and Müller traded the shorter time, 2 seconds, for a larger separation of the wave packets to a few microns, or a few thousandths of a millimeter. In each experiment, in a vacuum chamber, about 10,000 cesium atoms—too sparsely spaced to interact with each other—are dispersed by an optical grating into clouds of about 10 atoms.
“Gravity is trying to push them down with a force billions of times stronger than their attraction to the tungsten mass, but you have a return force from the optical grid holding them up, sort of like a shelf,” Panda said. “Then we take each atom and split it into two wave packets, so it’s now in a superposition of two pitches.” And then we take each of those two wave packets and put them in a separate grid, in a separate shelf, so it looks like a cabinet. When we turn off the grating, the wave packets recombine and all the quantum information that was acquired during the suspension can be read out.”
Panda plans to build his own lattice atom interferometer at the University of Arizona, where he has just been named an assistant professor of physics. He hopes to use it, among other things, to more precisely measure the gravitational constant, which relates the force of gravity to mass.
Meanwhile, Müller and his team are building a new lattice atom interferometer from scratch with better vibration control and lower temperature. The new device could produce results that are 100 times better than the current experiment, sensitive enough to detect the quantum properties of gravity. The planned experiment to detect gravitational entanglement, if successful, would resemble the first demonstration of quantum photon entanglement, performed at UC Berkeley in 1972 by the late Stuart Freedman and former postdoc John Clauser. Clauser shared the 2022 Nobel Prize in Physics for this work.
Reference: “Measurement of Gravitational Attraction with a Lattice Atom Interferometer” by Cristian D. Panda, Matthew J. Tao, Miguel Ceja, Justin Khoury, Guglielmo M. Tino, and Holger Müller, 26 Jun 2024, Nature.
DOI: 10.1038/s41586-024-07561-3
Other co-authors of the gravity paper include graduate student Matthew Tao and former undergraduate student Miguel Ceja of UC Berkeley, Justin Khoury of the University University of Pennsylvania in Philadelphia and Guglielmo Tino of the University of Florence in Italy. This work is supported by the National Science Foundation (1708160, 2208029), the Office of Naval Research (N00014-20-1-2656), and the Jet Propulsion Laboratory (1659506, 1669913).